In my latest blog post, I discussed how to read magnetograms and what we have to keep in mind regarding the Earth’s Magnetic Field and the location of the concerning site. Another important factor to approach the comprehensive interpretation of our data is the environment, esp. the geology, geomorphology and formation processes of the region. For magnetometry, it is especially the knowledge about magnetic properties (ferromagnetism) of rocks, minerals and soils.
The magnetogram shows the total magnetization, which is composed by the induced and the remanent magnetization (Fig. 1). The relation of induced and remanent magnetization is described by the Koenigsberger ratio (Q-ratio). It informs us not only about the quality of the rock sample for paleomagnetism, but also if we are dealing with archaeological objects.
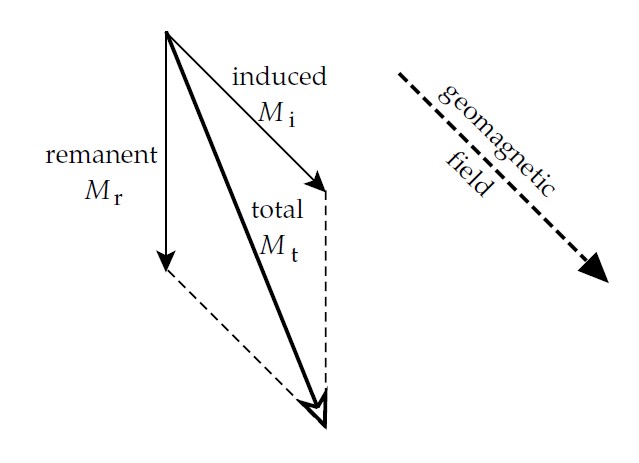
The induced magnetization exists with an applied external field only, e.g. the Earth’s Magnetic Field, and mostly goes along with the direction of the Earth’s Magnetic Field. For interpreting our magnetograms, it is helpful to conduct additional magnetic susceptibility measurements in the field, which tells us more about the induced magnetization. The magnetic susceptibility describes how magnetizable a sample/material is in an applied field. It is affected by the type of contained minerals as well as their grain size. The resulting values are unit less and can be negative (diamagnetic) or positive (ferromagnetic, ferrimagnetic, paramagnetic). Susceptibility can be measured in the field as well as in the laboratory, where more precise measurements are possible and additional parameter can be investigated.
The remanent magnetization is a permanent magnetization, independent of an external field, and important in paleomagnetism and archaeomagnetism. The natural remanent magnetization is the sum of the remanent magnetization and can be composed by several elements. For archaeological prospection, one of the most important remanent magnetizations is the thermoremanent magnetization (TRM). It is formed through heating of material over Curie temperature and cooling in an applied magnetic field, whose direction (Declination) is saved. Kilns, ovens and burnt objects like pottery or bricks etc. are the best examples for TRM. Chemical remanent magnetization (CRM) can be found in sedimentary or metamorphic rocks, whereas detrital remanent magnetization (DRM) develops during sedimentation of small magnetic particles in smooth water. Isothermal remanence (IRM) is the reason why we can detect also lightning strikes (LIRM) in our magnetograms. Although remanent magnetization is usually permanent, several factors could alter it, such as weathering.
But why are some materials/rocks more magnetic or magnetizable than others? It depends on iron oxides. Iron oxides are not only responsible for magnetization but also playing a role which colour a material has. The most important iron oxides regarding archaeological purposes are magnetite, maghemite, greigite, hematite, goethite as well as titanomagnetites, occurring in soils. While magnetite and maghemite are showing up to 1000 times higher susceptibilities than hematite, the latter is responsible for the typical red colour. Pedogenic, anthropogenic, lithogenic, and bacterial processes are responsible for the enhancement of soils, esp. top soils. Additionally, originally nonmagnetic materials can show enhanced magnetization: magnetotactic bacteria in organic materials are generating magnetite so that already gone posts, palisades etc. can be detected by magnetometry.
Magnetic susceptibility measurements in the field can be carried out selective e.g. for scattered objects and rocks on the surface or areal (separate geophysical prospection method). They can be used to define the extension of archaeological sites, activity zones or features in human made environments. Furthermore, they help understanding the morphology, formation processes, erosion and sedimentation as well as stratigraphic sequences for climate research and soil formation processes. Usually, top soils as well as archaeological soils are showing higher magnetic susceptibility values caused by enhancement of magnetic minerals due to the use of fire and fermentation. That is the reason why we can detect areas of human activity, e.g. settlements, and determine their extension.
How can we transfer this knowledge to the MUAFS concession area? The geological map of the Nile valley shows mostly sandstones, siltstones and mudstones accompanied by metavolcanic rocks as well as colluvium, sand sheets and dunes. For the volcanic rocks, we can assume high magnetic susceptibilities, whereas for the sandstones, siltstones and mudstones weak magnetic susceptibility is rather likely, depending on the contained minerals.
During the first geophysical campaign, we conducted spotty magnetic susceptibility measurements on our sites as well as the environment. Therefore, we used the handheld Kappameter SM-30 (Zh-instruments, Fig. 2). While magnetometry is a passive method, magnetic susceptibility meter are active instruments with a small coil included. Sampling the scattered rocks and archaeological objects like mudbricks gives an idea what we can expect in the magnetogram. Furthermore, the method can be applied in excavation trenches e.g. to distinguish stratigraphic layers or walls/floors and susceptibility maps can be produced. Due to magnetic susceptibility it is feasible to differentiate sources of raw material e.g. for mudbricks.
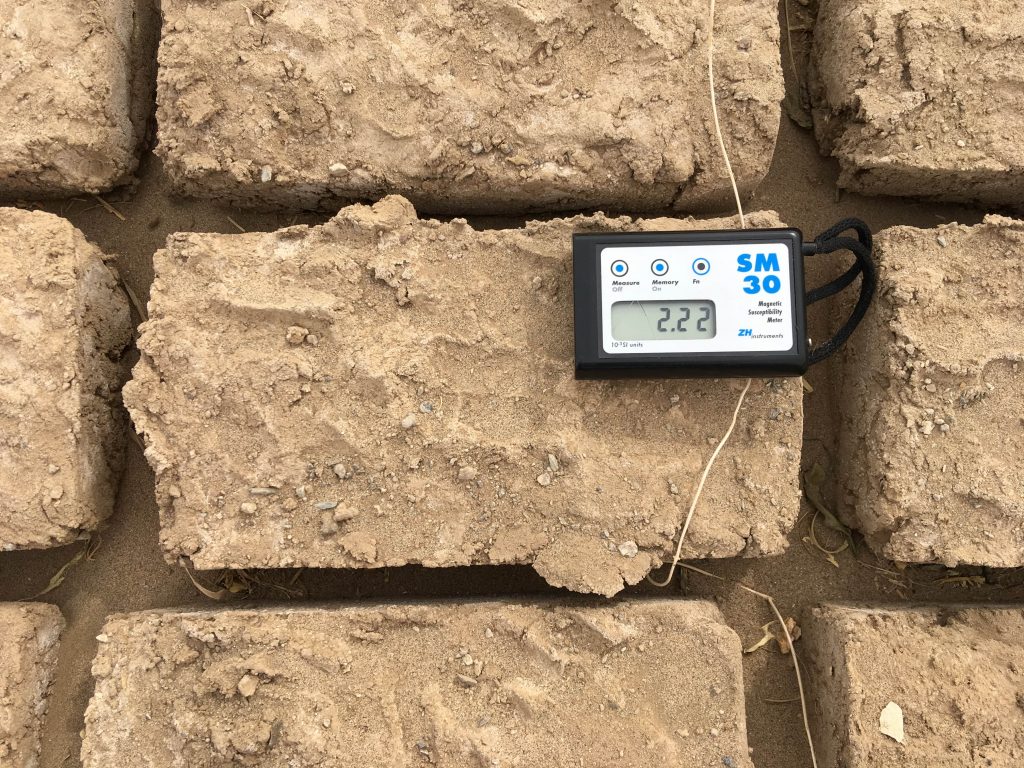
For the settlement site GiE 001 at Ginis East, the scattered rock material shows mostly susceptibility values in a range of 0,302 to 0,826 [10-3 SI], esp. quartz, schist, and sedimentary rocks, while rocks of volcanic origin result in values around 7,5 [10-3 SI]. The surface values are ranging between 4,35 to 5,6 [10-3 SI] and mudbrick from a partly upright standing hut shows values of around 4 [10-3 SI]. For this site, we could expect therefore the following: walls made of sedimentary rocks would cause negative anomalies in the magnetogram, while the use of volcanic rock would result in positive anomalies. Mudbrick or galus walls (of stamped mud) are more difficult to predict; depending on their mineral composition, they are revealing positive or negative anomalies. Fire installations or the use of fired bricks would be easily recognizable because of their high values.
A profile along a street close to the Nile River shows the different sedimentation layers very nicely: different colours as well as susceptibility values can be seen in Figure 3.
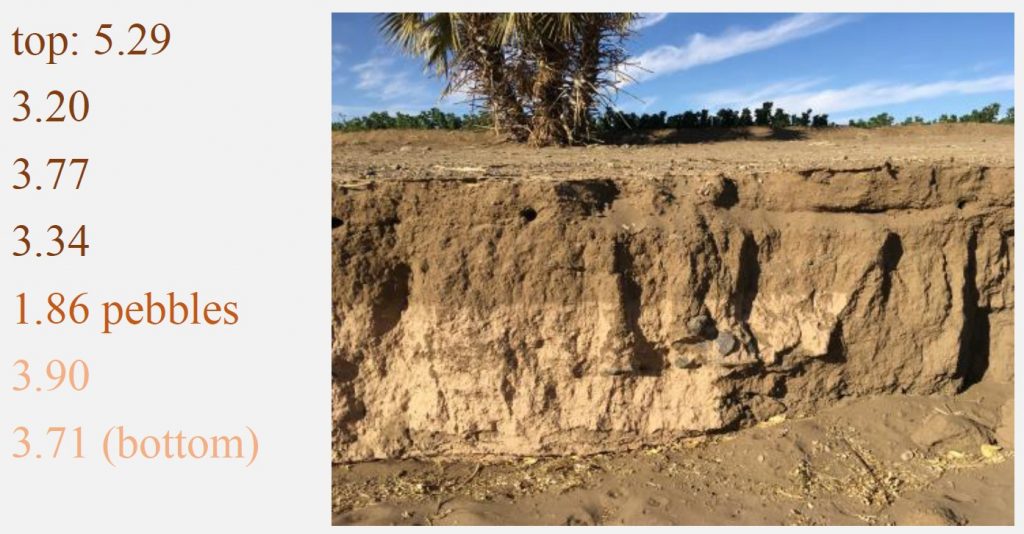
This example shows the complexity of magnetic susceptibility in combination with colours; darker and brighter layers show similar values, whereas the surface reaches the highest value. The layer with pebbles reveals the lowest value due to the included pebbles of probably sedimentary origin. For understanding the environment of archaeological sites and their formation processes, it is important to consider not only the survey, excavation and magnetometry results itself. Furthermore, knowledge in geology, geomorphology as well as the investigation of their parameters add details for a comprehensive picture of an archaeological landscape.
At the end, if you are asking yourself how magnetizable you are: without any ferromagnetic items from your clothes or e.g. glasses, the magnetic susceptibility would be almost zero or even negative, as the human body consists mostly of water.
References
Aspinall, A.; Gaffney, C.F.; Schmidt, A. (2008): Magnetometry for Archaeologists. Geophysical methods for archaeology 2. Lanham: AltaMira Press.
Butler, R.F. (1998): Paleomagnetism: Magnetic Domains to Geologic Terranes: Electronic Edition. Boston: Blackwell.
Dalan, R. (2017): Susceptiblity. In: Allan S. Gilbert, Paul Goldberg, Vance T. Holliday, Rolfe D. Mandel and Robert Siegmund Sternberg (eds.): Encyclopedia of Geoarchaeology. Dordrecht: Springer Reference (Encyclopedia of Earth Sciences Series), 939–944.
Fassbinder, J.W.E.; Stanjek, H.; Vali, H. (1990): Occurrence of Magnetic Bacteria in Soil. Nature 343 (6254), 161–163.
Fassbinder, Jörg W. E. (2017): Magnetometry for Archaeology. In: Allan S. Gilbert, Paul Goldberg, Vance T. Holliday, Rolfe D. Mandel and Robert Siegmund Sternberg (eds.): Encyclopedia of Geoarchaeology. Dordrecht: Springer Reference (Encyclopedia of Earth Sciences Series), 499–514.
Lowrie, W. (2007): Fundamentals of Geophysics, Cambridge: Cambridge University Press.